Spectroscopic Investigation
Uncover the mysteries of quantum interactions in groundbreaking research on ultrafast physics, terahertz spintronics, and meta-photonics—shaping the future of photonics technology.
Ultrafast Physics of Emerging Materials
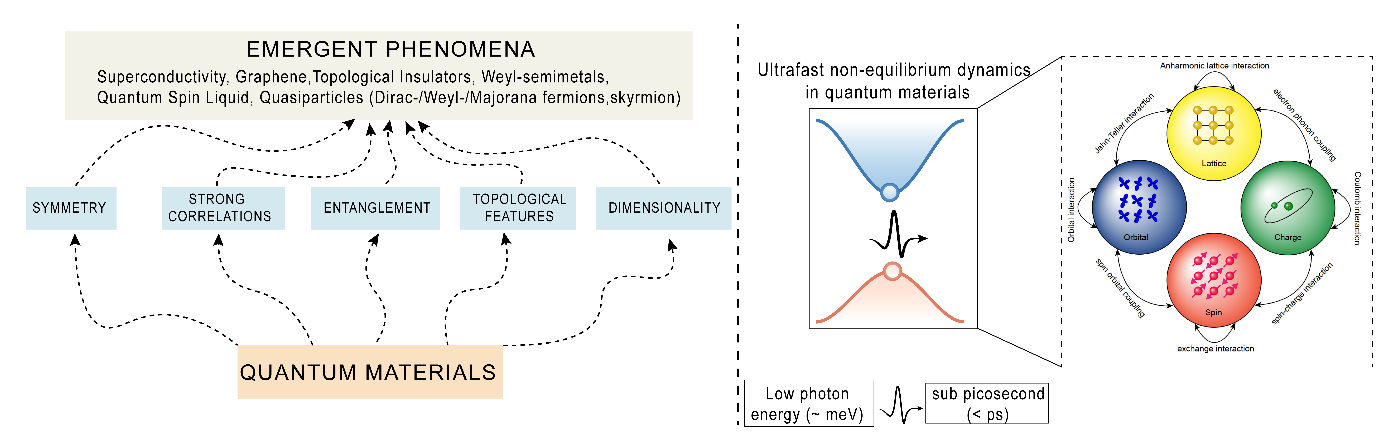
The term “quantum materials” is broad, encompassing a large portfolio of materials whose properties stem not only from correlation effects at microscopic level but also from the topological nature of the quantum wavefunctions. While all materials exhibit quantum mechanical properties to some level, ‘quantum materials’ showcase distinct features such as quantum fluctuations, quantum entanglement, quantum coherence, and topological behaviour (see Fig.1). Common examples of quantum materials include superconductors, topological insulators, Weyl semimetals and two-dimensional (2D) materials.
Interrogating the quantum materials using ultrafast spectroscopy techniques offer unique observables and capabilities to address some of the most pressing challenges in quantum materials. This spans from gaining a fundamental understanding of light–electron and light–spin interactions to unveiling hidden orders in equilibrium or excited states through unconventional light–matter interactions. Particularly, terahertz (THz) light, due to its low photon energy (a few electron volts) and sub picosecond time resolutions can interact resonantly or nob-resonantly with various degrees of freedom (see Fig. 1), driving many body phenomena. This capability enables the disentanglement of the role of electron, phonon, spin, and orbital degree of freedoms.
Ultrafast Terahertz Spintronics
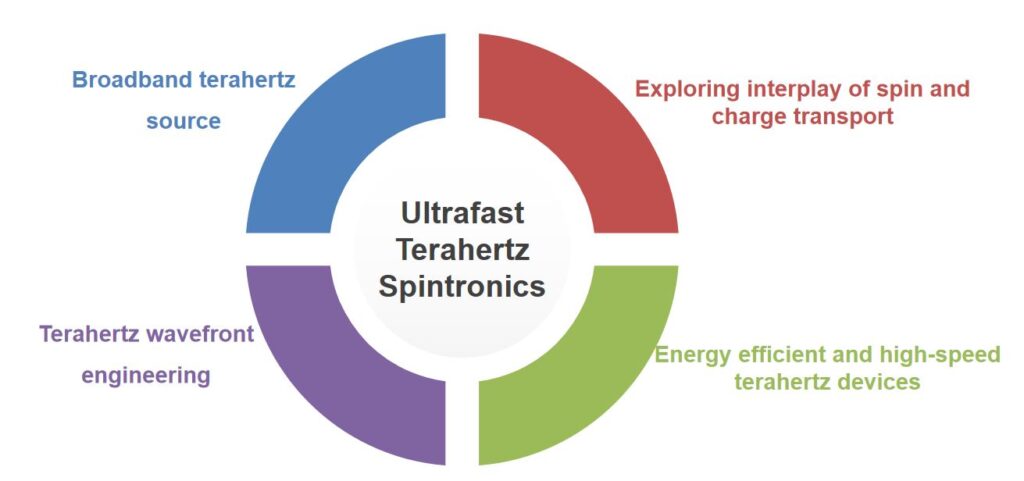
The primary objective of terahertz (THz) spintronics is to investigate and manipulate spin dynamics within spintronic systems using THz electromagnetic pulses. The exploration of spintronic structures using terahertz electromagnetic pulses, where 1 THz = Hz, proves to be a captivating pursuit for several reasons. Firstly, it provides a deeper comprehension of how electron spins interact with other degrees of freedom such as charge and orbitals, given that THz radiation directly engages with various fundamental modes at their inherent frequencies.
This interaction includes magnons, phonons, and intraband electron transport. Secondly, delving into the THz characteristics of key spintronic phenomena, such as spin-type Seebeck effects, offers insights into the initial stages of their development. Finally, by investigating THz spintronic effects, the potential for new applications emerges in both THz photonics (e.g., emitters and modulators of THz radiation) and spintronics (e.g., control and detection of THz spin dynamics).
Topological Light matter interactions
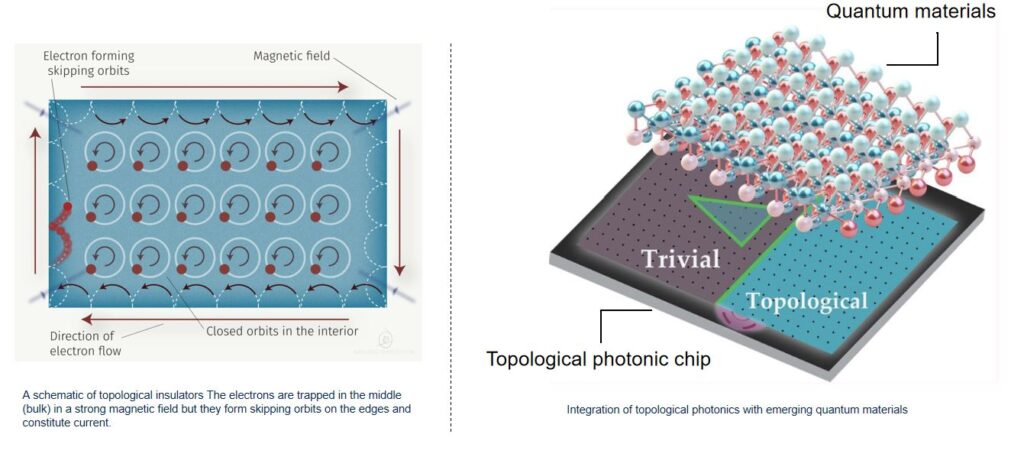
For many decades, physicists relied on the notion of symmetry breaking to distinguish different phases of matter. However, it has been recently shown that topology provides a universal way to classify the matter based on topological phases. The discovery of the topological phase of matter has revolutionized the understanding of solid-state physics. Their main hallmark is the appearance of edge states which are robust to backscattering at the interface between two materials of different topological phases (see above figure).
Recently, the concept of topology has been translated into the domain of photonics: topological photonics, with a grand vision to achieve robust and dissipation less transport of photons. We aim to study the interplay of topological light-matter interaction by combining topological edge states with a broad range of matter excitations such as excitons and phonons. Also, we are interested in integrating solid-state topological material systems with engineered photonic systems for realizing novel photonic devices.
Terahertz Induced Phase Transition
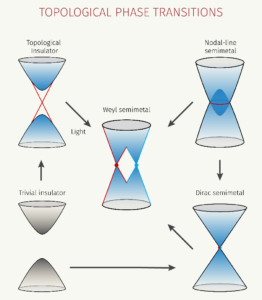
The interaction between light and matter in 2D and topological materials offers an intriguing means of manipulating emergent, non-equilibrium properties and unlocking novel functionalities within the ultrafast timescale, ranging from femtoseconds to picoseconds. In the last decade, noteworthy light-induced phenomena, including Bloch–Floquet states and photo-induced phase transitions, have been reported.
Through the resonant excitation of electrons or lattices, particularly with high-power terahertz light the energy landscape of 2D and topological materials can be changed. This alteration can give rise to light-induced phase transitions, such as the emergence of light-induced superconductivity or hidden states.